Apr 6, 2022
Irrigating to Replenish Plant-Available Water
Considering everything we do to grow a crop in Arizona, besides perhaps selecting the variety of the crop to be grown, irrigation is clearly the most important agronomic (crop and soil) aspect of crop management. The fact that Arizona consistently produces the highest yields and quality of crops found anywhere is a testimony to the fact that Arizona growers understand this quite well and they are extremely good at managing irrigation.
Part of what has made Arizona farmers so productive is the constant quest to improve on our irrigation methods, particularly in terms of improving irrigation efficiency. We know there are costs agronomically, economically, and environmentally associated with over- or under-irrigation and we constantly strive to alleviate both forms of irrigation water loss and improve irrigation efficiencies.
Irrigation is often considered as an engineering event and that it is true regarding the conveyance of water from a source and getting it to the field. However, the management of irrigation in the field, and particularly the management of both the timing and amount of irrigation, is completely an agronomic decision and action because the objective is to replenish the plant-available water in the soil for the benefit of the crop.
We have access to many methods and technologies to help improve irrigation management and efficiency (Cotton Inc. Sensor-based scheduling; Ratliff et al., 1983). Irrespective of the technologies or methods being used, all successful methods of irrigation management must deal with two basic issues: 1) tracking crop water use and 2) the replenishment of plant-available water in the soil with an irrigation event (Allen et al., 1988; Datta et al., 2017; Hanson et al., 2000).
For efficient management of irrigation water, there are several aspects of the total water in a soil that are important to recognize and the primary water fraction of interest is referred to as the “plant-available soil-water” (PAW). Figure 1 provides a graphic illustration of three important components of soil water: 1) total water in the soil (TW), 2) PAW, and 3) and unavailable soil-water to plants.
A very simple way to describe PAW in a soil can begin by considering the soil with a “sponge” analogy. If we put a sponge under a water faucet and fully saturate it, then let it hang in suspension, we will see free water draining from the sponge for a short period of time. We can consider a soil profile similarly after an irrigation where the soil will saturate and there will be drainage of the water that the soil cannot physically hold, this is referred to as drainage water, the leaching fraction, or the “gravitational water” (GW). The TW water is retained in the soil after the drainage water has moved out of the wetted zone. The TW is held in the soil due to the matrix forces of the soil particles that adhere to that water, like the forces holding water in the saturated sponge analogy. The TW remaining in the soil after the GW has drained away, contains the PAW and the non-available water that the soil holds by matrix forces stronger than what the plant extract (Figures 1 and 2).
There are physical definitions to describe several critical levels of soil-water content and the forces holding water in the soil. For example, field capacity (FC) is the point at which all the GW water has drained out of a soil. Soil physicists have defined FC as a function of potential energy at -33 kPa (kilopascals). Similarly, the permanent wilting point (PWP) has been defined as the point at which all PAW has been extracted from a soil. The PWP is defined somewhat arbitrarily at a potential energy level of -1500 kPa. The more negative the potential energy value, the lower the soil water content and the stronger the water is held in thin layers by the soil particles.
For practical field management of soil water, it is important to focus on the PAW for each soil and crop. It is important to understand that plants are not all created equal in terms of their capacity to extract water from a soil and some plants can extract more water from a given soil than other plants. For example, native desert plants (xerophytic plants) can extract much more water from a soil than most crop plants. Compared to native desert plants, our crop plants are much more sensitive. Therefore, the PAW illustrated in Figures 1, 2, and 3 are general descriptions but they illustrate an important soil-plant relationship. Each plant species has its own capacity to extract water from the soil and we need to know and understand this characteristic of each crop that we are managing in the field as well as the water holding capacity of the soil. For example, lettuce and most leafy-green vegetable crops are more sensitive to water stress than crops like cotton, wheat, melons, alfalfa, sorghum, etc. Thus, leafy green vegetable crops must be maintained at a higher level of PAW to avoid water stress in contrast to some other crop plants such as cotton, wheat, etc. The PWP for these crops will occur much before the soil-water content reaches the point of -1500 kPa.
Crop plants will draw upon the PAW fraction to sustain themselves physiologically and manage against water stress. Crop demands for water change as a function of stage of growth and environmental or weather conditions. Our fundamental irrigation management goal is to monitor the depletion of PAW in the soil and schedule an irrigation at the proper time and rate to replenish the PAW before the crop goes into water stress. That means we also need to identify the critical level of PAW for the crop in the field to determine when an irrigation is needed so we can avoid water stress on the crop.
The fact that different soil types, determined by soil texture, have different total and PAW holding capacities is well illustrated in Figure 1 and Table 1. Thus, it is important to know the soil type prevalent in any field to gauge the amount of PAW that a soil can hold. This will help determine to what extent the soil-water can be depleted and the amount of irrigation water needed to replenish the PAW.
There are many methods and technologies to measure soil-water and PAW to assist in irrigation management. However, the most fundamental method is to sample a soil and estimate “plant-available” water status by “feel”, by literally holding the soil in your hand and estimating the amount of plant-available water that is present.
A good way to calibrate the feel method is to check the soil in a field a few hours after an irrigation event when the GW has drained away and the soil is at FC. The next critical point to identify by the feel method is the soil-water content just before the plants begin to show any sign of water stress. The difference between those two points is the PAW that we are managing in the field. That critical point in the soil-water content that we need to recognize for managing irrigations for crop plants will still have a significant amount of PAW left in the soil but that is because the goal in crop management is to avoid plant water stress, which is signaled by the plant with wilting. Thus, that critical point for irrigating most crop plants is to do so just before the plant begins to experience water stress and show any sign of wilting.
To determine the moisture level in the soil we need to dig down with a shovel, soil probe, or with a soil auger to at least a depth of about 6-12 inches to get a representative soil sample. In general, for many crops and certainly vegetable crops, if the soil forms a tight ball and leaves a wet outline on your hand when you squeeze it, we can delay irrigation until the ball of soil, while still slightly moist and cool to the touch, is dry enough that it begins to crumble at the edges. The depth and time of irrigation should be long enough to fill the entire root zone of the plant, which will be dependent on both the soil texture (Table 1) and the level of soil water depletion.
Tracking and managing PAW water in the soil is a fundamental acquired skill that serves as a check on the irrigation scheduling methods or technologies being used and it remains an extremely valuable skill for anyone managing irrigations. We have many technologies available for irrigation management but a farmer or agronomist’s ability to recognize and understand these relationships and develop the necessary field skills is fundamental to good crop management. Identifying critical points in soil water content and plant growth, such as FC, PAW, and PWP by sampling a soil and making good estimates in the field by watching the crop and noting the various stages in crop development, serve a farmer or agronomist as a good standard and check on any technology being employed for irrigation management.
Farmers and agronomists learn to watch a crop in the field and recognize very subtle changes in leaf color and condition and they can also recognize the crop response and relationship to soil water content. That gives them the capacity to anticipate the optimum timing for the next irrigation. Farmers and agronomists understand these relationships among crop plants, soil water content, and the critical points for irrigation management. Irrigation management is an example of the blending of art and science that goes into good crop management, and it is certainly important in Arizona agriculture.
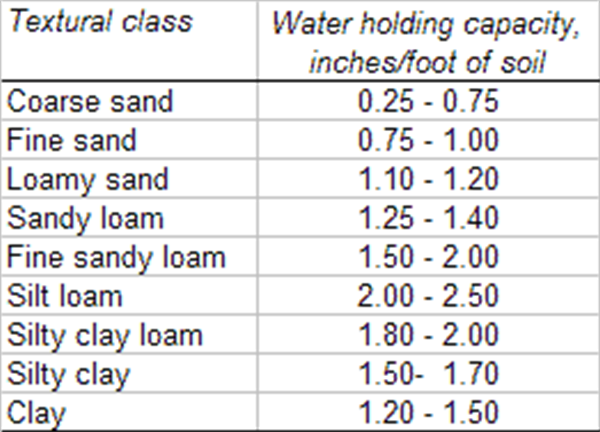
Table 1. Soil texture and water holding capacity.

Figure 1. Soil volume, soil texture, and water holding capacity relationships.

Figure 2. Soil water content relationship to plant-available water.
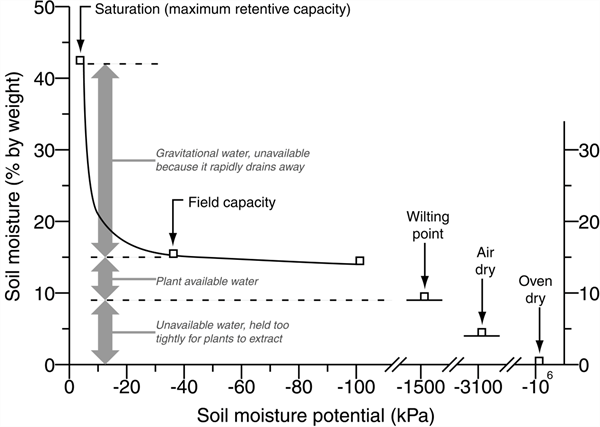
Figure 3. Soil moisture classes and important points on the soil moisture relationship curve. (Kansas State University Agronomy Department, Soil Laboratory Manual, soil and water relationships.)
References:
Allen, R. G., Pereira, L. S., Raes, D., & Smith, M. (1998). Crop evapotranspiration-Guidelines for computing crop water requirements-FAO Irrigation and drainage paper 56. FAO, Rome, 300(9), D05109.
Cotton Incorporated. Sensor-Based Scheduling. Retrieved from
http://www.cottoninc.com/fiber/AgriculturalDisciplines/Engineering/Irrigation-Management/Sensor-Based-Scheduling/
Datta, S., S. Taghvaeian, and J. Stivers. 2017. Understanding Soil Water Content and Thresholds for Irrigation Management. Oklahoma Cooperative Extension Service, BAE-1537
Hanson, B., Orloff, S., & Peters, D. (2000). Monitoring soil moisture helps refine irrigation management. California Agriculture, 54(3), 38-42.
Kansas State University Agronomy Department, Soil Laboratory Manual, soil and water relationships. https://kstatelibraries.pressbooks.pub/soilslabmanual/chapter/soil-and-water-relationships/
Ratliff, L., Ritchie, J., & Cassel, D. (1983). Field-measured limits of soil water availability as related to laboratory-measured properties. Soil Science Society of America Journal, 47(4), 770-775.